Whose Scientific Principle Explains The Lift In An Airplane Wing?
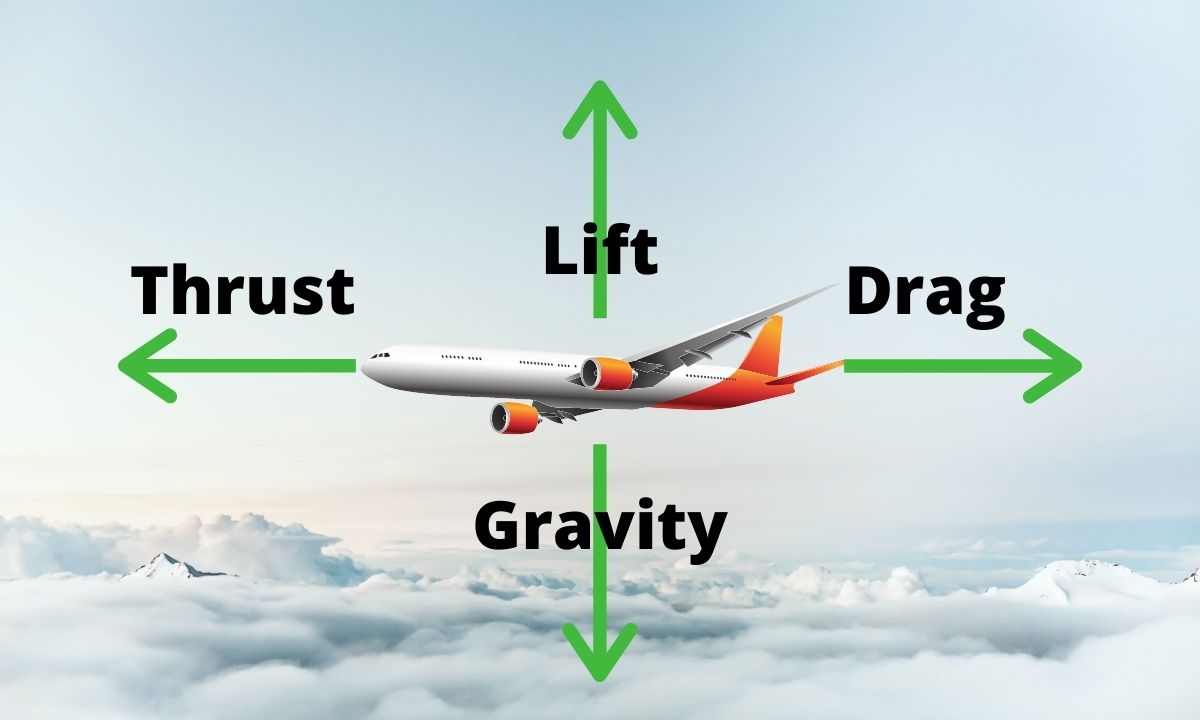
Introduction
When it comes to air travel, understanding the principles of flight is crucial. One of the most important concepts to grasp is the phenomenon of lift, which allows airplanes to soar through the sky. But have you ever wondered why an airplane wing is shaped the way it is, or what scientific principle allows it to generate lift?
In this article, we will explore the scientific principles behind the lift in an airplane wing. We’ll delve into the well-known Bernoulli’s principle and its role in generating lift. We’ll also discuss other factors, such as the Coanda effect and the angle of attack, that contribute to lift. By the end, you’ll have a better understanding of how airplanes stay airborne.
Whether you’re a frequent traveler, an aviation enthusiast, or just curious about the mechanics of flight, this article will provide you with a comprehensive overview of the essential concepts that underpin lift in an airplane wing.
Understanding Lift
Before we dive into the scientific principles behind lift, let’s first establish a basic understanding of what lift is. Lift is the force that opposes the weight of an airplane and allows it to stay in the air. Without lift, an airplane would simply plummet to the ground.
The concept of lift can be a bit counterintuitive. It might seem logical to assume that an airplane stays aloft because of its engines or the pushing force of the air. While these factors are indeed important, they are not the primary sources of lift. Instead, lift is primarily generated by the wings of an airplane.
As air flows over and under the wings, it creates different pressures that result in an upward force – lift. The shape and design of the wings are strategically engineered to maximize lift and minimize drag, allowing the airplane to achieve and sustain flight.
Now that we have a general understanding of what lift is, let’s dig deeper into the scientific principles that explain how it is generated in an airplane wing.
Bernoulli’s Principle
To understand the role of Bernoulli’s principle in generating lift, we need to examine the relationship between the speed of airflow and the pressure it creates. Bernoulli’s principle states that as the speed of a fluid increases, its pressure decreases, and conversely, as the speed decreases, the pressure increases.
In the context of airplane wings, the upper surface of the wing is curved while the lower surface is relatively flat. As a result, the airflow moving over the curved upper surface has to travel a longer distance and thus must move faster than the airflow underneath the wing. According to Bernoulli’s principle, this faster-moving airflow creates lower pressure on the upper surface compared to the lower surface.
The pressure difference between the upper and lower surfaces of the wing creates lift. This is because the higher pressure underneath the wing pushes upward against the lower pressure above the wing, creating an upward force. This force is what allows the airplane to overcome its weight and stay airborne.
It’s important to note that while Bernoulli’s principle plays a significant role in generating lift, it is not the sole factor responsible for keeping an airplane in the air. Other factors, such as the Coanda effect and the angle of attack, also contribute to the overall lift generation.
Now that we have a grasp of Bernoulli’s principle and its relationship to lift, let’s explore it in more detail to get a clearer understanding of the phenomena at play.
Explanation of Bernoulli’s Principle
To further understand how Bernoulli’s principle contributes to lift generation, let’s break it down into simpler terms. As mentioned earlier, the airflow over the curved upper surface of the wing moves faster than the airflow under the flat lower surface. This difference in airflow speed results in a difference in pressure.
When the air moves faster over the wing’s curved upper surface, it has to cover a greater distance in the same amount of time compared to the airflow under the wing. As a result, the air molecules become more spread out, resulting in lower air pressure. On the other hand, the slower-moving air underneath the wing remains relatively compressed, leading to higher air pressure.
The pressure difference between the upper and lower surfaces generates lift. The lower pressure above the wing creates an upward force, while the higher pressure underneath the wing contributes to the overall lift by pushing against the lower pressure. This pressure differential creates a net force that overcomes the weight of the airplane and keeps it in the air.
It’s crucial to note that Bernoulli’s principle is not limited to just airplane wings. The same principle applies to a wide range of fluid dynamics, including water flow through pipes, air movement in wind tunnels, and even the flight of birds. Bernoulli’s principle is a fundamental concept in understanding the dynamics of fluid flow and its applications in various fields.
While Bernoulli’s principle elegantly explains lift generation, it is important to acknowledge that it is a simplified representation of the complex aerodynamics involved in flight. The understanding and explanation of lift continue to be refined and expanded upon as scientists and engineers explore the intricacies of flight.
Criticisms and Alternatives
While Bernoulli’s principle provides a useful framework for understanding lift generation, it has faced some criticisms and alternative explanations over the years. Let’s explore some of these criticisms and alternative theories that shed light on the complexities of lift.
One criticism of Bernoulli’s principle is that it oversimplifies the physics of lift. It fails to account for the effects of airflow separation, turbulence, and the three-dimensional nature of wing aerodynamics. In reality, the generation of lift in an airplane wing is a combination of multiple factors, including pressure distribution, airflow circulation, and vortices.
One alternative theory that challenges the exclusivity of Bernoulli’s principle is the concept of Newtonian flow. According to Newtonian flow theory, lift is primarily a result of downward deflection of the airflow due to the angle of attack of the wing. This theory emphasizes the role of the wing’s shape and angle in redirecting the airflow and generating lift.
Another alternative explanation is found in the circulation theory proposed by Kutta and Joukowski. This theory suggests that lift is mainly generated through the creation of a circulation or vortex around the wing. The circulation produces a pressure difference between the upper and lower surfaces, creating lift in a manner similar to Bernoulli’s principle.
It’s worth noting that these alternative explanations are not necessarily in contradiction with Bernoulli’s principle, but rather provide complementary perspectives. Lift generation is a complex interaction of various factors, and each theory contributes to our understanding of the phenomenon.
In recent years, computational fluid dynamics (CFD) and advanced wind tunnel testing have allowed researchers to obtain more accurate and detailed insights into the complexities of lift generation. These advancements have enabled engineers to design more efficient and aerodynamically optimized wings, further pushing the boundaries of flight performance.
While Bernoulli’s principle remains an essential concept in explaining lift generation, it’s important to recognize that it is just a part of the broader picture. Understanding the complexities and interplay of various aerodynamic factors enhances our knowledge of flight and contributes to the continuous improvement of aviation technology.
The Coanda Effect
In addition to Bernoulli’s principle, another important factor contributing to lift generation is the Coanda effect. The Coanda effect describes the tendency of a fluid, such as air, to adhere to the surface of a curved object rather than follow a straight path.
When it comes to airplane wings, the curvature of the upper surface causes the airflow to stay attached to the wing, even as the wing moves through the air. This phenomenon allows the air to “stick” to the wing’s surface for a longer distance, resulting in an increased pressure difference between the upper and lower surfaces.
By utilizing the Coanda effect, the wings of an airplane can generate more lift. The curved upper surface of the wing helps redirect the airflow downwards, increasing the pressure difference and providing an additional upward force.
The Coanda effect is particularly crucial during takeoff and landing, when the angle of attack is high. In these situations, the airflow separation and the creation of vortices can significantly affect lift generation. The Coanda effect helps delay airflow separation by keeping the air attached to the wing’s surface, allowing for a more efficient and stable lift production.
It’s important to note that while the Coanda effect plays a significant role in lift generation, it’s not the sole factor. The combination of Bernoulli’s principle, the Coanda effect, and other aerodynamic factors work together to create the necessary lift for flight.
Researchers and engineers continue to study and refine our understanding of the Coanda effect and its implications for lift generation. This knowledge helps inform the design and optimization of airplane wings, improving efficiency, stability, and overall flight performance.
The Angle of Attack
The angle of attack is a critical parameter in determining lift generation in an airplane wing. The angle of attack refers to the angle between the wing’s chord line (an imaginary line connecting the leading and trailing edges of the wing) and the oncoming airflow.
When the angle of attack increases, the wing’s relative airflow increases as well, resulting in higher lift. This is because a higher angle of attack creates a greater pressure difference between the upper and lower surfaces of the wing. As a result, more lift is generated to counteract the weight of the airplane.
However, it’s important to note that there is an optimal angle of attack for maximum lift. Beyond this optimal point, the airflow over the wing becomes turbulent and separates from the surface, leading to a decrease in lift and an increase in drag. This phenomenon, known as stalling, can cause the airplane to lose control and potentially lead to a dangerous situation.
Pilots carefully control the angle of attack during different phases of flight to optimize lift and maintain safe and stable flight. During takeoff and landing, a higher angle of attack is typically used to generate maximum lift and ensure a safe touchdown. During cruise flight, a lower angle of attack is employed to minimize drag and improve fuel efficiency.
It’s crucial for pilots and designers to consider the balance between lift and drag when determining the optimal angle of attack for a specific aircraft. Through aerodynamic testing, computer simulations, and real-world flight data, engineers can refine wing designs and optimize the angle of attack to achieve the best possible performance.
In summary, the angle of attack is a key parameter in lift generation. By adjusting the angle of attack, pilots can control the amount of lift generated by the wings, ensuring safe and efficient flight. Understanding the effects of the angle of attack is essential for both pilots and aircraft designers in creating and maintaining reliable and high-performance aircraft.
Other Factors Affecting Lift
While Bernoulli’s principle, the Coanda effect, and the angle of attack are key contributors to lift generation, there are several other factors that significantly impact an airplane wing’s ability to produce lift. Let’s explore some of these additional factors:
1. Wing Shape: The shape of the wing plays a crucial role in lift generation. Wings are designed with specific airfoil shapes, both in terms of their curvature and thickness, to optimize lift and minimize drag. Different aircraft have different wing designs based on their intended purpose, such as high-speed flight or efficient cruising.
2. Wing Area: The surface area of the wing directly affects the amount of lift generated. A larger wing surface area provides more surface area for air to interact with, resulting in greater lift. Aircraft designed for heavy lifting, like cargo planes, often have larger wings to generate the necessary lift to carry heavy loads.
3. Wing Loading: The wing loading refers to the weight supported by a unit of wing area. Higher wing loading means more weight is supported by a smaller wing area, which can affect lift generation. High wing loading may require higher speeds to generate sufficient lift, while low wing loading allows for lower speeds and improved maneuverability.
4. Airspeed: The speed at which an aircraft travels through the air affects the lift produced by the wings. As airspeed increases, the airflow over the wing accelerates, creating a larger pressure difference and generating more lift. Pilots must consider the appropriate airspeed for different phases of flight to maintain the desired lift and control.
5. Air Density: The density of the air also plays a crucial role in lift generation. Higher density air provides more air molecules for the wing to interact with, resulting in increased lift. Changes in altitude can have a significant impact on air density, requiring pilots to adjust their flying techniques accordingly.
6. Flaps and Slats: Flaps and slats are movable surfaces located on the trailing edge and leading edge of the wing, respectively. These devices can be extended or retracted to modify the shape of the wing during takeoff, landing, and important maneuvers. By changing the wing’s shape, flaps and slats can increase lift production and improve aircraft control.
By understanding and harnessing these factors, engineers and pilots can optimize lift generation and maximize the performance of an aircraft. The interplay of these variables allows for safer, more efficient flight and enables aircraft to fulfill their intended roles effectively.
Conclusion
Understanding the principles behind lift generation in airplane wings is crucial for anyone interested in aviation or simply curious about how airplanes stay airborne. While Bernoulli’s principle serves as a fundamental concept, it is just one piece of the puzzle. Lift generation is a complex interplay of factors, including the Coanda effect, the angle of attack, wing shape, airspeed, air density, and the use of flaps and slats.
By carefully designing and engineering airplane wings, experts can optimize lift production, minimize drag, and improve overall flight performance. Pilots, on the other hand, utilize their skill and knowledge to control the angle of attack and airspeed to maintain safe and stable flight.
Advancements in computational fluid dynamics and wind tunnel testing continue to enhance our understanding of lift generation, leading to the development of more efficient and innovative aircraft designs. The quest for improved performance and safety drives ongoing research and innovation in the field of aerodynamics.
In conclusion, lift generation is a fascinating and intricate process that allows airplanes to defy gravity and soar through the sky. The combination of scientific principles, engineering expertise, and piloting skill creates the perfect balance required to keep airplanes airborne and passengers safely traveling to their destinations. So the next time you’re flying high above the clouds, take a moment to appreciate the marvels of lift and the intricate science that makes it all possible.