What Controls The Pitch Of An Airplane
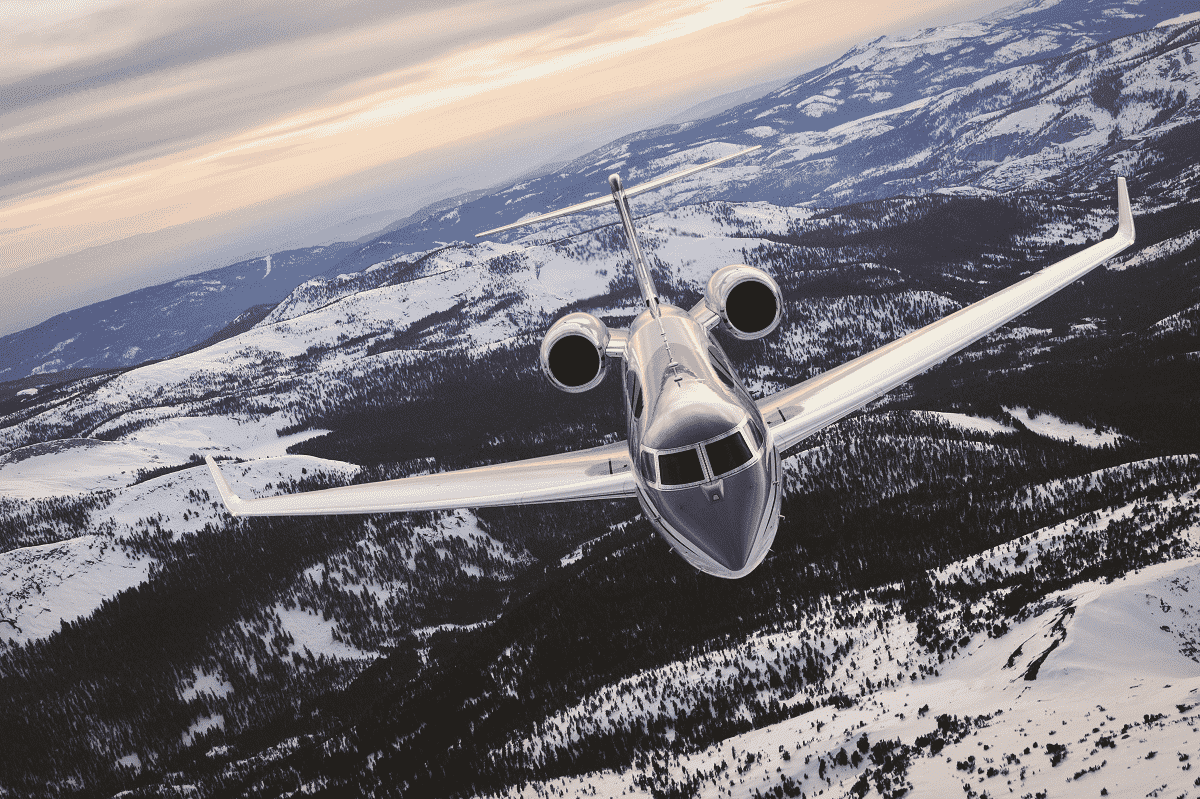
Introduction
When it comes to air travel, one of the most critical aspects of flight control is the ability to control the pitch of an airplane. Pitch control refers to the up and down motion of the aircraft’s nose, which determines its angle of ascent or descent. Essentially, it is what allows the airplane to climb, descend, or maintain a level flight.
To control the pitch of an airplane, various systems and components work together seamlessly, allowing pilots to maneuver the aircraft with precision and responsiveness. Understanding the fundamentals of pitch control is crucial for both aviation enthusiasts and frequent flyers who want to gain insight into the intricacies of flight.
In this article, we will explore the basics of pitch control, the key components involved, and how they interact to influence the aircraft’s pitch. From manual control systems to cutting-edge fly-by-wire technology, we’ll delve into the mechanisms that enable pilots to maintain the desired pitch during flight.
So, whether you’re curious about the inner workings of an airplane or simply want to enhance your knowledge of aviation, join us as we demystify the world of pitch control in aircraft.
The Basics of Pitch Control
Pitch control is crucial for maintaining stability and controlling the altitude of an aircraft. Understanding the basics of pitch control is essential for safe and efficient flight. At its core, pitch control is the ability to control the aircraft’s nose angle, which determines whether the aircraft climbs, descends, or maintains level flight.
To manipulate the pitch of an aircraft, pilots rely on various control surfaces and mechanical systems. The primary control surface responsible for pitch control is the elevator, located on the horizontal stabilizer at the tail of the airplane. By adjusting the elevator’s position, pilots can alter the amount of lift generated by the aircraft’s wings, thus controlling the pitch.
When the pilot wants to pitch the nose up, they pull back on the control column or joystick, which deflects the elevator upwards. This changes the airflow over the horizontal stabilizer and increases the aircraft’s pitch angle. Conversely, when the pilot wants to pitch the nose down, they push the control column or joystick forward, deflecting the elevator downwards and decreasing the aircraft’s pitch angle.
It’s important to note that pitch control goes hand in hand with two other critical controls: roll and yaw. Roll control refers to the side-to-side rotation of the aircraft around its longitudinal axis, while yaw control involves the rotation around its vertical axis. These three control axes (pitch, roll, and yaw) work together to provide complete control over the aircraft’s movements.
Proper pitch control is crucial for maintaining a safe and stable flight. If the nose of the aircraft is pitched too high, it can lead to a stall where the wings lose lift and the aircraft loses altitude rapidly. On the other hand, if the nose is pitched too low, it can result in excessive speed, compromising the aircraft’s control and safety.
Next, we will dive deeper into the elevator and its function in pitch control, shedding light on the details of this critical control surface and how it contributes to the aircraft’s maneuverability.
The Elevator and its Function
When it comes to pitch control, the elevator plays a central role in maneuvering the aircraft. The elevator is a control surface located on the horizontal stabilizer at the tail of the airplane, and it is responsible for controlling the aircraft’s pitch.
The elevator functions by changing the lift generated by the wings. When the pilot moves the control column or joystick, it adjusts the elevator’s position, causing it to deflect either up or down. This deflection changes the angle of the airflow over the horizontal stabilizer and therefore alters the distribution of lift on the wings.
When the elevator is deflected downwards, it increases the angle of attack of the tailplane. This creates more lift on the tail and pushes the tail of the aircraft down, causing the nose to pitch up. Conversely, when the elevator is deflected upwards, it decreases the angle of attack of the tailplane, reducing the lift on the tail and allowing the nose to pitch down.
The amount of elevator deflection determines the degree of pitch change. Small adjustments in elevator deflection result in subtle changes in pitch, while larger deflections can produce more significant pitch changes. Pilots adjust the elevator deflection to control the pitch angle and maintain the desired flight path.
The elevator is typically connected to the control column or joystick in the cockpit through a system of cables, pushrods, or hydraulic systems. When the pilot moves the control input, it transmits the corresponding signal to the elevator, causing it to move accordingly.
It’s worth mentioning that modern aircraft, especially commercial jetliners, often utilize fly-by-wire technology for pitch control. In these systems, electronic signals are sent to actuators that move the elevator, replacing traditional mechanical linkages. Fly-by-wire systems offer several advantages, including lighter weight, improved precision, and enhanced control redundancy.
Now that we understand the elevator’s function in pitch control, let’s explore the role of the horizontal stabilizer, the structure that houses the elevator and contributes to the aircraft’s stability.
The Role of the Horizontal Stabilizer
The horizontal stabilizer, also known as the tailplane, is an essential component of an aircraft’s pitch control system. Located at the tail of the airplane, it serves several crucial functions in maintaining stability and controlling the aircraft’s pitch.
One of the primary roles of the horizontal stabilizer is to provide stability by counteracting the pitching moment generated by the wings during flight. As the wings generate lift, they also create a pitching moment that tries to rotate the aircraft around its lateral axis. The horizontal stabilizer resists this moment and helps maintain the aircraft’s longitudinal stability.
The elevator, as we discussed earlier, is attached to the horizontal stabilizer. It is the control surface that moves up and down to control the aircraft’s pitch. The horizontal stabilizer enhances the efficiency and effectiveness of the elevator by providing a lever arm. This lever arm increases the mechanical advantage of the elevator, allowing it to exert more control force on the aircraft’s pitch.
Another crucial role of the horizontal stabilizer is to provide a surface for the elevator to attach to. It serves as the structure that houses the elevator, connecting it to the rest of the aircraft. The horizontal stabilizer provides stability and strength to the elevator, ensuring that it can function properly and withstand the aerodynamic forces during flight.
The size and design of the horizontal stabilizer can vary depending on the aircraft’s type and intended purpose. Larger aircraft with higher payloads and speeds tend to have larger horizontal stabilizers to provide the necessary stability and control authority. The shape and angle of the horizontal stabilizer also contribute to the overall aerodynamic characteristics of the aircraft.
In some aircraft, particularly those with low-speed flight capabilities, additional control surfaces called trim tabs may be present on the trailing edge of the horizontal stabilizer. These trim tabs can be adjusted to fine-tune the aircraft’s pitch and relieve the pilot of constantly holding pressure on the control column or joystick.
By working in conjunction with the elevator, the horizontal stabilizer ensures that the aircraft maintains stable and controlled pitch throughout various flight conditions. With the understanding of the elevator’s function and the role of the horizontal stabilizer, we can now explore the impact of other control surfaces on pitch control.
Control Surfaces and their Effect on Pitch
In addition to the elevator and horizontal stabilizer, there are other control surfaces on an aircraft that play a role in pitch control. These control surfaces, when manipulated by the pilot, can produce changes in the aircraft’s pitch behavior.
One such control surface is the aileron, which is located on the wings near the trailing edge. The primary function of the aileron is to control the aircraft’s roll, but it also has a secondary effect on pitch. When the pilot operates the ailerons, they create a differential lift between the wings, causing the aircraft to roll. This rolling motion can indirectly affect the pitch by altering the airflow over the wings, leading to changes in lift distribution and, consequently, the pitch attitude of the aircraft.
Another control surface that affects pitch is the canard. The canard is a small forward wing-like structure located near the nose of some aircraft. It operates in a similar manner to the main wing, generating lift. By adjusting the canard’s position, pilots can directly influence the aircraft’s pitch. When the canard is deflected downwards, it generates additional lift and causes the nose to pitch up. Conversely, when the canard is deflected upwards, it decreases lift and allows the nose to pitch down.
Flaps and slats, located on the wings’ trailing and leading edges, respectively, are also control surfaces that can impact pitch. While their primary function is to increase lift and improve low-speed flight performance, the deployment of flaps and slats can lead to changes in the aircraft’s pitch. When the flaps and slats extend, they alter the airflow over the wings, affecting the lift distribution and potentially causing the aircraft’s nose to pitch up or down.
It’s important to note that the effect of these control surfaces on pitch may vary depending on the aircraft’s design and configuration. Different aircraft types and models have unique aerodynamic characteristics that influence the extent to which these control surfaces impact pitch control.
Pilots must have a thorough understanding of how these control surfaces interact to achieve the desired pitch response. By properly manipulating these control surfaces, pilots can adjust the aircraft’s pitch to maintain stability, perform maneuvers, and safely navigate through different flight phases.
Having explored the various control surfaces and their impact on pitch control, next, we will delve into another crucial factor that influences pitch: weight and balance.
The Influence of Weight and Balance on Pitch
Weight and balance are crucial considerations in aviation, and they have a significant impact on an aircraft’s pitch behavior. The distribution of weight and maintaining the correct balance are essential for safe and stable flight.
The center of gravity (CG) is a key factor in weight and balance. The CG is the point where the aircraft’s weight is considered to be concentrated. It is determined by the distribution of passengers, cargo, fuel, and other items on board. The CG location affects the aircraft’s stability, maneuverability, and pitching characteristics.
When the CG is too far forward, the aircraft becomes nose-heavy. This configuration can result in a tendency for the nose to pitch down, making it more challenging for the pilot to maintain level flight. Conversely, when the CG is too far aft, the aircraft becomes tail-heavy, leading to a tendency for the nose to pitch up. This can affect the aircraft’s control responsiveness and stability.
To maintain proper weight and balance, aircraft manufacturers provide recommended limits for CG location. Pilots must ensure that the weight distribution falls within these limits to ensure safe and predictable flight characteristics. They can adjust the distribution of passengers, cargo, and fuel to achieve the desired CG position.
Furthermore, changes in weight and balance during flight can also affect pitch control. As fuel is consumed, the aircraft’s weight decreases, causing a shift in the CG position. Pilots must be aware of these changes and make necessary adjustments to maintain the desired pitch attitude.
It’s important to note that weight and balance considerations extend beyond the individual flight. Proper loading and distribution of weight can affect the long-term structural integrity of the aircraft. Overloading or improper weight distribution can put excessive stress on the airframe, compromising its strength and safety.
By carefully managing weight and balance, pilots can ensure that the aircraft maintains optimal pitch control characteristics, improving stability, control responsiveness, and overall flight safety.
Having explored the influence of weight and balance on pitch, we will now delve into the aerodynamic forces that contribute to pitch control.
Aerodynamic Forces and Pitch Control
Aerodynamic forces are the driving factors behind an aircraft’s ability to maintain pitch control. Understanding these forces is essential for pilots to effectively manage the aircraft’s pitch behavior.
There are two principal aerodynamic forces at play in pitch control: lift and weight. Lift is the upward force generated by the wings as they move through the air. It counters the force of gravity, which we refer to as weight. The relative magnitudes of lift and weight determine whether an aircraft climbs, descends, or maintains level flight.
By increasing or decreasing the angle of attack, pilots can alter the amount of lift the wings generate, and consequently, the amount of upward or downward force on the aircraft. Raising the angle of attack results in increased lift, causing the aircraft to pitch up. Lowering the angle of attack reduces lift, leading to a pitch-down motion.
Another important aerodynamic force in pitch control is drag. Drag is the resistance encountered by an aircraft as it moves through the air. It acts opposite to the direction of motion and affects the aircraft’s speed and energy. Drag also plays a role in pitch control, particularly when it comes to managing the speed and stability of the aircraft.
Additionally, the location of the center of pressure (COP) on the aircraft’s wings is critical in pitch control. The COP is the point where the average aerodynamic forces act on the wings. If the COP is located ahead of the CG, it creates a nose-down moment, while a COP located behind the CG creates a nose-up moment. By adjusting the aircraft’s configuration and control surfaces, pilots can manipulate the location of the COP and influence the pitching forces accordingly.
It is important for pilots to carefully manage these aerodynamic forces during flight to achieve the desired pitch control. By understanding how lift, weight, drag, and the center of pressure interact, pilots can manipulate the aircraft’s pitch to maintain stability, perform maneuvers, and respond to changing flight conditions.
Now that we have explored the aerodynamic forces involved in pitch control, let’s discuss the mechanisms and systems that enable pilots to physically control the aircraft’s pitch.
Mechanical Systems for Pitch Control
Mechanical systems play a crucial role in enabling pilots to control the pitch of an aircraft. These systems provide the physical means for pilots to manipulate the control surfaces and adjust the aircraft’s pitch attitude.
One of the most common mechanical systems for pitch control is the conventional cable and pulley system. In this system, cables run from the control column or joystick in the cockpit to the elevator control surfaces at the tail of the aircraft. When the pilot moves the control input, the cables transmit the corresponding motion to the elevator, causing it to deflect and adjust the pitch attitude of the aircraft.
Another mechanical system used in larger and more complex aircraft is the pushrod system. In this system, instead of cables, rigid rods or tubes are used to transmit the control inputs from the cockpit to the control surfaces. These pushrods push or pull the control surfaces, such as the elevator, to change the aircraft’s pitch.
Hydraulic systems are also utilized in some aircraft for pitch control. Hydraulic actuators provide the necessary force to move the control surfaces. When the pilot operates the control input, hydraulic pressure is sent to the actuators, which move the control surfaces accordingly and adjust the pitch of the aircraft.
As aircraft have become more advanced, fly-by-wire technology has gained popularity. Fly-by-wire systems use electronic signals instead of physical linkages to transmit control commands. In these systems, the pilot’s control inputs are converted into electrical signals and transmitted to actuators that move the control surfaces. Fly-by-wire systems offer greater precision, flexibility, and the ability to incorporate advanced control algorithms.
Additionally, mechanical systems for pitch control often incorporate mechanisms to provide feedback to the pilot. For example, control columns and joysticks are designed with mechanical force feedback, allowing pilots to sense and adjust their input based on the resistance felt. This tactile feedback enhances the pilot’s situational awareness and helps maintain precise control over the aircraft’s pitch.
These mechanical systems for pitch control undergo rigorous testing and maintenance to ensure their reliability and effectiveness. Regular inspections, lubrication, and adjustment are carried out to guarantee that the control systems operate smoothly and respond accurately to pilot inputs.
Now that we have explored the mechanical systems for pitch control, let’s examine the advancements in technology that have revolutionized the way pilots manipulate and control the aircraft’s pitch.
Fly-by-Wire Technology and Pitch Control
Fly-by-Wire (FBW) technology has revolutionized aircraft control systems, including pitch control. FBW replaces the traditional mechanical linkages between the pilot’s control inputs and the control surfaces with electronic signals and computer-based systems, offering numerous advantages in terms of reliability, flexibility, and performance.
One of the key benefits of FBW technology in pitch control is its ability to provide precise and responsive control of the aircraft’s pitch. With FBW, pilot inputs are converted into electrical signals that are processed by a flight control computer. The computer then adjusts the control surfaces, such as the elevator, to achieve the desired pitch attitude. This direct electronic control eliminates any mechanical play and allows for highly accurate and consistent pitch control.
Another advantage of FBW technology is its flexibility in adapting to different flight conditions. The flight control computer can incorporate various control laws and algorithms that automatically adjust the pitch response based on factors like airspeed, load factors, and stability requirements. This adaptability enhances the aircraft’s handling characteristics and improves flight safety.
FBW systems also offer redundancy and fault tolerance. Multiple flight control computers can operate in parallel, cross-checking each other’s inputs and outputs. If a fault is detected in one computer, the others can take over and ensure continued pitch control. This redundancy enhances the reliability and resilience of the aircraft’s control systems, reducing the risk of complete control failure.
Moreover, FBW technology enables advanced features like envelope protection and stability augmentation. The flight control computer continuously monitors the aircraft’s flight parameters and limits the pilot’s control inputs to ensure they don’t exceed safe limits. This envelope protection helps prevent stalling, overspeeding, or extreme maneuvers that could compromise the aircraft’s stability and control.
Additionally, FBW technology allows for the integration of other control functions and automation systems, such as auto-trim and autopilot. These systems assist pilots in maintaining the desired pitch attitude and relieving them of continuous manual control inputs, enhancing workload management and reducing pilot fatigue.
With the advancement of FBW technology, the pilot’s control inputs are now transmitted through electronic interfaces, including control sidesticks, which provide tactile feedback and communicate the aircraft’s behavior. This haptic feedback ensures that pilots remain connected and aware of the aircraft’s pitch response, even in the absence of physical linkages.
In summary, Fly-by-Wire technology has revolutionized pitch control by replacing traditional mechanical systems with electronic signals and flight control computers. It offers precise control, adaptability to various flight conditions, redundancy, and additional safety features. This technology has significantly improved the efficiency, safety, and overall performance of modern aircraft.
Now, let’s conclude our exploration of pitch control in aircraft.
Conclusion
Pitch control is a fundamental aspect of aviation that allows pilots to maintain stability, perform maneuvers, and safely navigate through different flight conditions. In this article, we have explored the key components and mechanisms involved in pitch control, from the elevator and horizontal stabilizer to the various control surfaces and aerodynamic forces.
The elevator, located on the horizontal stabilizer, plays a crucial role in controlling the aircraft’s pitch by adjusting the lift distribution on the wings. Other control surfaces, such as ailerons, canards, flaps, and slats, also impact pitch indirectly through their influence on lift distribution and airflow over the wings.
We have also discussed the influence of weight and balance on pitch control, emphasizing the importance of maintaining the proper distribution of weight to achieve optimal stability and control responsiveness. Changes in fuel consumption during flight can significantly impact the aircraft’s weight and balance, necessitating adjustments to maintain the desired pitch attitude.
Aerodynamic forces, including lift, weight, drag, and the location of the center of pressure, contribute to pitch control. By manipulating these forces through changes in angle of attack and adjusting control surfaces, pilots can control the aircraft’s pitch and maintain the desired flight path.
Mechanical systems, such as cable and pulley systems, pushrod systems, and hydraulic systems, provide the physical means for pilots to manipulate the control surfaces and adjust pitch. However, the advent of Fly-by-Wire technology has revolutionized pitch control with its precise electronic control, adaptability, redundancy, and advanced features like envelope protection and stability augmentation.
Understanding pitch control is essential not only for pilots but also for aviation enthusiasts who strive to comprehend the complex dynamics of flight. By grasping these concepts, we can appreciate the intricate systems and mechanisms that enable safe and efficient air travel.
In conclusion, pitch control is a critical aspect of aviation that ensures the stability, performance, and safety of aircraft. Through a combination of mechanical systems, aerodynamic forces, and technological advancements, pilots are equipped with the tools necessary to navigate the skies with precision and confidence.