How High Can A Airplane Fly
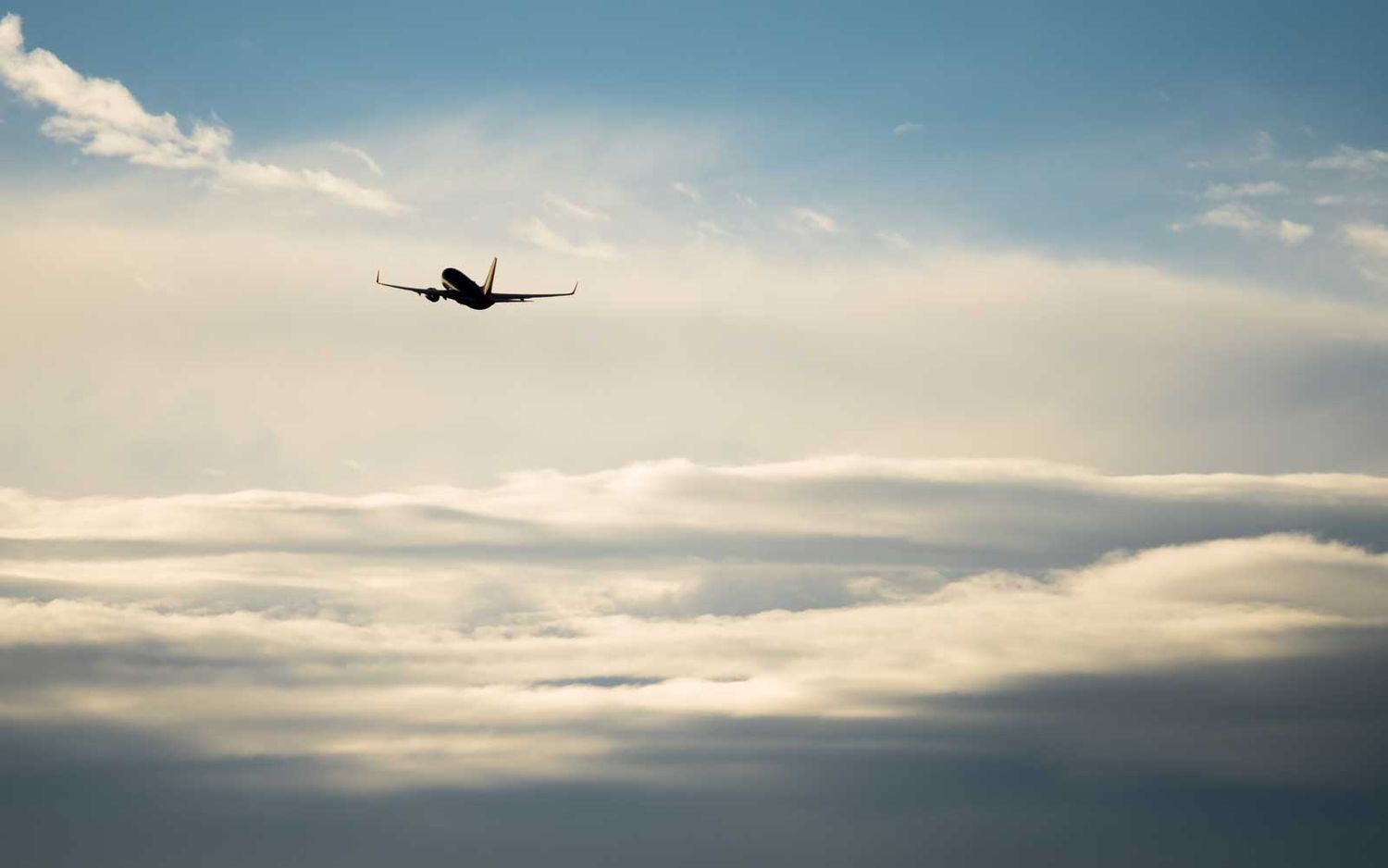
Introduction
When it comes to air travel, one of the fascinating aspects is how high airplanes can soar in the sky. From the moment they leave the ground to the altitude they reach during long-haul flights, airplanes have the remarkable ability to navigate through the vast expanse of the atmosphere. Understanding the factors that influence the maximum altitude an airplane can fly is not only intriguing but also crucial for pilots, aircraft designers, and aviation enthusiasts.
The maximum altitude an airplane can reach depends on various factors, including engine performance, airplane design, crew limitations, atmospheric conditions, and technological advancements. These factors work together to determine the optimal altitude for a safe and efficient flight. By exploring these factors, we can gain a deeper understanding of the capabilities and limitations of airplanes when it comes to flying at high altitudes.
In this article, we will delve into the world of aviation and explore the factors that influence the maximum altitude of airplanes. From the performance limitations of engines to the structural constraints of aircraft design, we will examine how these elements shape the altitude capabilities of different aircraft. Additionally, we will discuss the role of oxygen supply and crew limitations when operating at high altitudes, as well as the impact of atmospheric conditions on flight altitude. Lastly, we will explore the highest recorded altitudes reached by various types of airplanes and look ahead to future innovations that may push the boundaries even further.
So buckle up and prepare for a high-flying adventure as we explore the fascinating world of airplane altitudes and the factors that determine just how high they can go.
Factors Influencing the Maximum Altitude of Airplanes
The maximum altitude an airplane can reach is influenced by a multitude of factors. These factors can be broadly classified into engine performance and altitude limitations, airplane design and structural limitations, oxygen and crew limitations, and atmospheric conditions. Let’s dive into each of these factors to gain a better understanding of their impact on flight altitude.
Engine Performance and Altitude Limitations: The power and performance of an airplane’s engines play a significant role in determining its maximum altitude. As an airplane climbs higher, the air density decreases, resulting in reduced engine performance. This decrease in air density affects the engine’s ability to produce thrust, which in turn limits the aircraft’s ability to climb to higher altitudes. However, advancements in engine technology, such as more efficient turbofan engines, have increased the altitude capabilities of modern aircraft.
Airplane Design and Structural Limitations: The design and structure of an airplane also impact its maximum altitude. The aerodynamic properties of the aircraft, including its wingspan, wing area, and wing curvature, play a crucial role in determining its ability to generate lift at higher altitudes. Additionally, the structural integrity of the airplane must be taken into account when considering altitude limitations. At extreme altitudes, the reduced air pressure and temperature can put stress on the aircraft’s structure, necessitating careful design considerations.
Oxygen and Crew Limitations at High Altitudes: As an airplane climbs to higher altitudes, the oxygen levels in the atmosphere decrease significantly. To ensure the safety and well-being of the crew and passengers, aircraft are equipped with pressurized cabins that maintain a breathable environment. However, there are limits to the altitude at which pressurization is effective. Beyond these limits, supplemental oxygen is required for both the crew and passengers. Additionally, prolonged exposure to high altitudes can lead to physiological challenges, such as altitude sickness, which must be carefully managed.
Impacts of Atmospheric Conditions on Maximum Altitude: Atmospheric conditions, including temperature, wind patterns, and air density, can have a significant impact on the maximum altitude an airplane can reach. Cold temperatures at higher altitudes can affect engine performance and increase fuel consumption. Wind patterns can cause turbulence and affect the stability of the aircraft at extreme altitudes. Furthermore, variations in air density due to factors such as altitude and weather conditions influence an airplane’s ability to generate lift and maintain steady flight.
By taking into account these factors, pilots and aircraft designers can optimize flight performance and ensure the safety and efficiency of air travel at different altitudes. It is the careful balance between these factors that determines the maximum altitude an airplane can reach, balancing the capabilities of the aircraft with the limitations imposed by physics and human physiology.
Engine Performance and Altitude Limitations
The engine performance of an airplane plays a crucial role in determining its maximum altitude capabilities. As an aircraft climbs higher into the atmosphere, the air density decreases, which directly affects engine performance. Understanding the relationship between engine performance and altitude limitations is essential for pilots and aircraft designers alike.
When an airplane’s engines operate at a higher altitude, the reduced air density leads to a decrease in the amount of oxygen available for combustion. This reduction in oxygen content directly impacts the engine’s power output. It becomes more challenging for the engine to generate the necessary thrust to maintain altitude or climb further.
Engine manufacturers design engines with specific altitude limits in mind. These limitations are based on factors such as the type of engine, design specifications, and intended usage. Turbine engines, commonly used in commercial aircraft, have higher altitude capabilities compared to reciprocating engines found in smaller propeller-driven aircraft.
Advancements in engine technology, such as the development of turbofan engines, have contributed to improved altitude capabilities. Turbofan engines are more efficient at higher altitudes, thanks to their ability to compress and cool the air before it enters the combustion chamber. This allows for better fuel combustion and thus improved engine performance.
Engine performance at high altitudes is also influenced by ambient air temperature. Cold temperatures at high altitudes can affect engine performance in several ways. Firstly, cold air is denser, which can result in increased engine power output. However, extremely low temperatures can also cause issues such as ice formation on critical engine components, leading to reduced engine efficiency and potential malfunctions.
It’s important to note that the altitude capabilities of an aircraft’s engines are not solely determined by engine design. Factors such as aircraft weight, wing design, and other aerodynamic considerations contribute to overall performance. These factors directly impact an airplane’s ability to generate the required lift to climb to higher altitudes.
In aviation, engine performance and altitude limitations are closely monitored and considered during flight planning and operations. Pilots must ensure that the chosen altitude is within the capabilities of the aircraft’s engines. This involves considering factors such as temperature, aircraft weight, intended flight path, and air traffic control regulations.
Engine performance and altitude limitations are key factors that pilots and aircraft designers must understand to ensure safe and efficient flight operations. By working closely with engine manufacturers and employing advanced technologies, the aviation industry continues to push the boundaries of altitude capabilities, enabling airplanes to reach higher altitudes with improved performance and safety.
Airplane Design and Structural Limitations
The design and structure of an airplane play a critical role in determining its maximum altitude capabilities. From wing design to overall aerodynamics, various factors must be considered to ensure the aircraft’s structural integrity and optimal performance at high altitudes.
One of the key considerations in airplane design is the wingspan and wing area. The wings generate the lift necessary to keep the airplane airborne. As an aircraft climbs to higher altitudes, the reduced air density puts increased demand on the wings to generate lift. A longer wingspan and larger wing area allow for more efficient lift production, enabling the aircraft to reach higher altitudes with improved performance.
In addition to wingspan and area, the curvature of the wings, also known as the wing camber, plays a crucial role in determining an airplane’s altitude capabilities. The shape of the wing affects how the air flows over and beneath it, creating the necessary lift. Optimal wing camber ensures efficient airflow, reducing drag and allowing the aircraft to maintain stability and reach higher altitudes.
The structural integrity of an airplane is also a vital consideration when it comes to altitude limitations. At higher altitudes, the air pressure decreases, putting stress on the aircraft’s structure. Engineers need to ensure that the materials used and the overall design are capable of withstanding the decreased air pressure and potential temperature variations. Failure to consider these factors could lead to structural failures or compromises in safety.
Furthermore, the weight of the aircraft plays a significant role in determining maximum altitude capabilities. A heavier airplane will require more lift at higher altitudes, which puts additional strain on the wings and other structural components. Aircraft designers employ various techniques, such as lightweight materials and efficient design principles, to reduce weight and optimize altitude performance.
Modern advancements in materials science and design techniques have enabled the development of aircraft with improved structural integrity and lighter weight. Carbon fiber composites and other high-strength materials are now commonly used in aviation to create stronger yet lighter structures, increasing the altitude capabilities of modern airplanes.
It’s worth mentioning that airplane design and structural limitations are not only important for reaching higher altitudes but also for maintaining stability and performance at all flight levels. Aircraft must be designed to handle various flight conditions, including turbulence and extreme weather, while ensuring passenger comfort and safety.
In summary, the design and structural limitations of an airplane significantly impact its maximum altitude capabilities. Factors such as wingspan, wing area, wing camber, and overall structural integrity all play integral roles in determining an aircraft’s ability to reach higher altitudes. By continuously refining design principles and leveraging advanced materials, aircraft designers strive to push the boundaries of altitude performance while ensuring safety and efficiency in aviation.
Oxygen and Crew Limitations at High Altitudes
As airplanes climb to higher altitudes, the decrease in atmospheric oxygen levels becomes a significant consideration for both the crew and passengers. Oxygen supply and crew limitations are crucial factors to ensure the safety and well-being of everyone on board.
At higher altitudes, where the air is less dense, the concentration of oxygen molecules in the atmosphere decreases. As a result, the partial pressure of oxygen reduces, which can lead to hypoxia, a condition caused by insufficient oxygen supply to body tissues. To mitigate the effects of hypoxia, commercial aircraft are equipped with pressurized cabins that maintain a higher air pressure, providing a breathable environment for passengers and crew.
Pressurized cabins can maintain a comfortable and safe breathing environment up to certain altitudes, typically around 8,000 to 10,000 feet. However, beyond this altitude, additional oxygen supplementation becomes necessary to ensure adequate oxygen levels in the blood. This is particularly crucial for crew members who need to remain alert and perform their duties effectively.
Pilots and crew members are trained to recognize the signs of hypoxia and the need for supplemental oxygen. They have access to onboard oxygen systems that supply oxygen masks for emergency use. These masks allow crew members to receive a direct flow of oxygen at higher altitudes, ensuring their safety and ability to perform their duties under such conditions.
It’s worth noting that for passengers, the effects of reduced cabin pressurization are typically less severe, thanks to the relatively short duration of flights. However, in situations where flights reach very high altitudes or extended durations, supplemental oxygen systems may be available for passenger use.
In addition to the physical effects of altitude on oxygen levels, crew limitations also need to be considered. Aviation regulations dictate the maximum amount of time a crew member can spend at high altitudes before requiring rest. These limitations are in place to prevent fatigue and ensure the crew’s ability to make sound decisions and operate the aircraft safely. Crew members are required to have adequate rest and may not be allowed to operate flights at high altitudes if they have exceeded their allowable duty hours.
Furthermore, prolonged exposure to high altitudes can result in physiological challenges for crew members. Altitude sickness, characterized by symptoms such as headaches, dizziness, and nausea, can impact crew performance and safety. It is crucial for crew members to acclimatize to higher altitudes gradually and be aware of the signs and symptoms of altitude-related illnesses.
In summary, oxygen supply and crew limitations are paramount at high altitudes. Pressurized cabins, supplemental oxygen systems, and crew rest regulations ensure the safety and well-being of pilots, crew members, and passengers. By adhering to these limitations and monitoring oxygen levels, airlines can effectively manage the challenges posed by high altitudes and ensure a comfortable and secure travel experience for all.
Impacts of Atmospheric Conditions on Maximum Altitude
Atmospheric conditions have a profound impact on the maximum altitude an airplane can reach. Factors such as temperature, wind patterns, and air density all play a significant role in the aircraft’s performance and its ability to achieve higher altitudes. Let’s explore how these atmospheric conditions influence flight altitude.
Temperature: Temperature variations in the atmosphere can affect the performance of an airplane. As an aircraft ascends to higher altitudes, the temperature typically decreases. Cold temperatures can have both positive and negative impacts. On one hand, colder air is denser, resulting in increased engine performance and enhanced lift generation. On the other hand, extremely low temperatures can cause critical components to freeze, impairing the smooth operation of the aircraft. This delicate balance between temperature and performance must be carefully monitored to ensure safe and efficient flight operations.
Wind Patterns: The wind patterns at different altitudes can influence an airplane’s ability to reach its maximum altitude. Strong headwinds can increase the time and fuel required to climb to higher altitudes, as the aircraft must overcome the opposing forces. Tailwinds, on the other hand, can assist in reaching higher altitudes more quickly. Changes in wind speed and direction with altitude, known as wind shear, can also have significant effects on flight stability and control. Pilots must be aware of these wind patterns and make necessary adjustments to ensure a safe climb to higher altitudes.
Air Density: Air density plays a vital role in determining the maximum altitude an airplane can achieve. As an aircraft climbs to higher altitudes, the air density decreases. Reduced density means there are fewer oxygen molecules available for combustion in the engine, resulting in decreased engine performance and reduced thrust. This decrease in air density also affects the ability of the wings to generate sufficient lift. As a result, airplanes may experience reduced climb rates and require longer distances to reach higher altitudes.
Variations in air density due to changes in temperature and atmospheric pressure can impact an aircraft’s ability to maintain steady flight. Pilots use performance charts and take into account current weather conditions to calculate the optimal altitude for the aircraft. By understanding the relationship between air density and altitude, pilots can plan their flight path accordingly and achieve the desired altitude while ensuring safety and efficiency.
It’s crucial to note that atmospheric conditions are constantly changing and vary based on geographic location, time of year, and weather patterns. Pilots rely on up-to-date weather information and forecasts to make informed decisions about altitude selection and flight path adjustments. Additionally, modern aircraft are equipped with advanced weather radar systems and communication networks that provide real-time weather data, enabling pilots to navigate around severe weather conditions and optimize their performance at different altitudes.
In summary, atmospheric conditions have a significant impact on the maximum altitude an airplane can reach. Temperature variations, wind patterns, and air density all influence the aircraft’s performance and its ability to climb to higher altitudes. By carefully considering these factors and making necessary adjustments, pilots can navigate through the atmosphere safely and efficiently, reaching the desired altitudes while maintaining stability and control.
The Highest Recorded Altitude by Different Types of Airplanes
Aircraft have continually pushed the boundaries of altitude capabilities throughout aviation history. Different types of airplanes, from commercial airliners to military jets and specialized research aircraft, have achieved impressive altitudes. Let’s explore some notable examples of the highest recorded altitudes reached by various types of aircraft.
Commercial Airliners: Commercial airliners, such as the Boeing 747 and Airbus A380, typically operate at cruising altitudes of around 35,000 to 40,000 feet. However, the highest recorded altitude for a commercial airliner goes to the Concorde, which was capable of reaching altitudes of up to 60,000 feet. This supersonic aircraft, with its unique design and powerful engines, could cruise at speeds exceeding Mach 2 (twice the speed of sound) at such high altitudes.
Military Jets: Military jet aircraft often have higher altitude capabilities than commercial airliners due to their design and performance characteristics. The Lockheed U-2 spy plane holds the record for one of the highest altitudes achieved by a piloted aircraft. It can fly at altitudes of up to 70,000 feet, allowing it to conduct high-altitude reconnaissance missions.
Experimental and Research Aircraft: Specialized experimental and research aircraft have pushed the boundaries of altitude exploration. The NASA X-15 rocket plane holds the record for the highest altitude reached by a piloted, powered aircraft. During its flights in the 1960s, the X-15 climbed to altitudes above 350,000 feet, surpassing the edge of space. This remarkable achievement contributed valuable data to the field of high-speed flight and space exploration.
Unmanned Aerial Vehicles (UAVs): In recent years, unmanned aerial vehicles, or drones, have demonstrated impressive altitude capabilities. High-altitude, long-endurance drones, such as the NASA Helios Prototype and the Airbus Zephyr, have set records by reaching altitudes exceeding 60,000 feet. These drones can stay airborne for extended periods, performing tasks such as atmospheric research, communication relays, and surveillance.
Balloons and Airships: Although not technically airplanes, balloons and airships have achieved remarkable altitudes. The highest recorded altitude reached by a balloon goes to the helium-filled balloon piloted by Felix Baumgartner during the Red Bull Stratos mission in 2012. Baumgartner ascended to an altitude of approximately 128,100 feet (39,044 meters) before making a record-breaking freefall jump back to Earth.
These examples highlight the extraordinary altitude capabilities of different types of aircraft. While commercial airliners operate at altitudes that provide optimum efficiency and passenger comfort, specialized aircraft, such as military jets, experimental planes, and drones, have reached altitudes previously unimaginable. These achievements in altitude exploration continue to drive advancements in aviation technology and inspire new possibilities for future aircraft design and performance.
Challenges and Safety Considerations at Extreme Altitudes
Operating at extreme altitudes presents unique challenges and safety considerations for pilots and aircraft designers. As airplanes climb to higher altitudes, they encounter a range of factors that can impact flight performance, crew and passenger well-being, and overall safety. Let’s explore some of the challenges and safety considerations faced when operating at extreme altitudes.
Reduced Oxygen Levels: One of the primary challenges at extreme altitudes is the decreased availability of oxygen. As an airplane ascends, the air becomes thinner, resulting in lower oxygen levels. This can pose a significant risk to crew and passengers, as insufficient oxygen supply can lead to dizziness, impaired cognitive function, and, in severe cases, loss of consciousness. To mitigate this, aircraft are equipped with pressurized cabins and supplemental oxygen systems to maintain a breathable environment and ensure the safety of those on board.
Crew Fatigue and Performance: Prolonged exposure to high altitudes can lead to crew fatigue, impacting their performance and decision-making abilities. The reduced air pressure and oxygen levels can contribute to physiological effects such as reduced concentration, decreased reaction time, and decreased cognitive function. Crew members must be vigilant in managing their fatigue levels and take necessary rest breaks to ensure they can effectively perform their duties at extreme altitudes.
Temperature Extremes: Extreme altitudes are often accompanied by extreme temperature variations. At higher altitudes, temperatures can plummet, posing challenges for aircraft systems and components. Cold temperatures can affect the functionality of critical systems such as fuel lines, hydraulic systems, and electrical equipment. It is essential for aircraft designers to consider these temperature extremes and ensure that the aircraft’s systems are appropriately insulated and capable of operating in harsh environments.
Structural Limitations: Extreme altitudes put additional strain on an aircraft’s structure. The reduced air pressure at high altitudes can lead to structural stresses and challenges. It is crucial for aircraft designers to consider the structural integrity of the aircraft, ensuring that it can handle the decreased air pressure without compromising safety. This involves using strong and lightweight materials and employing robust engineering principles to ensure the aircraft’s structural stability at extreme altitudes.
Turbulence and Weather Conditions: Extreme altitudes can be associated with unique atmospheric conditions, including severe turbulence and challenging weather patterns. Strong winds and turbulence can impact the stability of the aircraft, making it crucial for pilots to remain alert and make necessary flight adjustments to maintain control. Additionally, thunderstorms and other severe weather conditions can pose risks at extreme altitudes, requiring pilots to carefully plan their flight paths and avoid turbulent or hazardous areas.
Overall, operating at extreme altitudes presents various challenges and safety considerations that must be carefully managed. From oxygen supply and crew fatigue to temperature extremes, structural limitations, and weather conditions, pilots and aircraft designers must prioritize safety and ensure the well-being of crew and passengers. Through comprehensive training, advanced aircraft systems, and careful planning, aviation professionals strive to overcome these challenges and ensure safe and efficient operations at extreme altitudes.
Future Innovations and Possibilities for Higher Flight Altitudes
The aviation industry continues to drive innovation and explore new possibilities for reaching higher flight altitudes. Advancements in technology, materials, and aircraft design open up exciting opportunities for future aircraft to operate at even more extreme altitudes. Let’s explore some of the potential future innovations for higher flight altitudes.
Hypersonic Flight: Hypersonic flight, involving speeds greater than Mach 5, is an area of promising innovation. Hypersonic aircraft would possess the ability to fly at extraordinarily high altitudes, potentially reaching the fringes of space. These futuristic aircraft would require advanced propulsion systems and heat-resistant materials capable of withstanding the intense heat generated due to air friction at such high speeds.
Spaceplanes: Another intriguing possibility is the development of spaceplanes that can seamlessly transition between Earth’s atmosphere and space. These vehicles would offer the potential to reach suborbital altitudes, allowing for rapid and efficient travel between continents. In the future, spaceplanes could revolutionize long-haul flights, drastically reducing travel times and opening up possibilities for space tourism.
Improved Engine Technology: Advancements in engine technology continue to enhance aircraft performance and altitude capabilities. The development of more efficient engines, such as advanced turbofans or hybrid-electric propulsion systems, could allow for increased thrust and improved fuel efficiency at high altitudes. This would enable aircraft to climb to higher altitudes more easily and potentially break altitude records.
Lightweight and Strong Materials: The use of lightweight and strong materials is an ongoing focus in aircraft design. Advanced composites, such as carbon fiber reinforced polymers, offer the potential for lighter and stronger structures. These materials can enable aircraft to withstand the challenges of extreme altitudes while also reducing weight, conserving fuel, and improving overall performance.
Improved Life Support Systems: To overcome the challenges of reduced oxygen levels at extreme altitudes, future aircraft may feature enhanced life support systems. These systems could include improved cabin pressurization technologies, smarter oxygen delivery systems, and advanced monitoring systems to ensure the well-being and safety of passengers and crew at high altitudes.
Autonomous and Unmanned Aircraft: The development of autonomous and unmanned aircraft opens up exciting possibilities for reaching higher flight altitudes. These advanced aircraft, operating without pilot limitations, could push the boundaries of altitude exploration. Unmanned high-altitude vehicles could be used for tasks such as atmospheric research, satellite deployment, or infrastructure inspections in challenging environments.
While these future innovations and possibilities for higher flight altitudes are exciting, they also come with challenges and considerations. Safety, environmental impact, regulatory frameworks, and infrastructure requirements are just some of the factors that must be addressed when exploring these new frontiers of aviation. However, with continued research, investment, and collaboration, the aviation industry is poised to reach new heights and redefine what is possible in the realm of flight altitudes.
Conclusion
The maximum altitude an airplane can fly is influenced by various factors, including engine performance, airplane design, crew limitations, atmospheric conditions, and technological advancements. Engine performance and altitude limitations go hand in hand, with advancements in engine technology pushing the boundaries of altitude capabilities. Airplane design and structural limitations play a crucial role in determining an aircraft’s maximum altitude, ensuring both aerodynamic efficiency and structural integrity. Oxygen supply and crew limitations at high altitudes are essential for ensuring the safety and well-being of both the crew and passengers. Atmospheric conditions, such as temperature, wind patterns, and air density, pose challenges and impact an aircraft’s ability to achieve higher altitudes. The highest recorded altitudes reached by different types of airplanes demonstrate the remarkable achievements in aviation history. Operating at extreme altitudes presents additional challenges and safety considerations, including reduced oxygen levels, crew fatigue, temperature extremes, structural limitations, and turbulence. However, future innovations and possibilities for higher flight altitudes continue to captivate the imagination of the aviation industry. Advances in technology, improved engine performance, lightweight materials, improved life support systems, and autonomous aircraft open up exciting opportunities for reaching even higher altitudes in the future. With continued research and development, the boundaries of flight altitudes can be further expanded, unlocking new possibilities for efficient, safe, and awe-inspiring air travel.